Super Critical Water Reactors (SCWR)
SuperCritical Water-Cooled Reactors (SCWRs) are advanced nuclear reactors operating at temperatures and pressures above water's critical point (374°C, 22.1 MPa). SCWRs can feature thermal, fast, or mixed neutron spectra and are designed in two configurations: pressure vessel (similar to BWRs and PWRs) and pressure tubes (like CANDU reactors). Combining design insights from existing water-cooled reactors and supercritical fossil-fired plants, SCWRs achieve higher thermal efficiencies of 44-48%, significantly improving over the current 34-36%. These reactors offer economic benefits through increased efficiency and simpler designs. Representing an evolutionary step from existing technologies, SCWRs have yet to be built or operated.
Presentation of the Super Critical Water Reactor System
SuperCritical Water-Cooled Reactors (SCWRs) are advanced water-cooled nuclear reactors operating above the thermodynamic critical point of water (i.e. 374°C, 22.1 MPa). SCWRs can operate under different neutron spectra, such as thermal, fast, or mixed neutron, depending on the core design. There are two main core configurations proposed for SCWR concepts: one is based on light water reactor (LWR) technology using a large pressure vessel (e.g., Boiling Water Reactors (BWR) and Pressurized Water Reactors (PWR), and the other is based on a calandria vessel and pressure tubes (e.g., CANDU reactors).
The various SCWR concepts rely on the proven design technology and operation experience gained through the operation of hundreds of water-cooled reactors and experience from supercritical fossil-fired plans. Supercritical fluids are common in the power industry and have been used for more than 70 decades in the energy sector. The first supercritical boiler was built in 1960. The reason for using supercritical fluids in the energy sector is due to the enhanced thermodynamic efficiency of the system, which means the same amount of energy produced, but with less fuel consumed. For example, SCWRs aim to reach higher thermal efficiency (44-48% or more) than the current water-cooled reactors (34-36 %).
Furthermore, supercritical fluids have a unique characteristic: They behave as a single-phase fluid, allowing for simpler designs, such as a thermodynamic direct cycle. Several SCWR concepts opted for this cycle. Selecting a direct cycle reduces the number of components in the plant, thus reducing the plant's initial costs and potentially increasing the plant's reliability as no steam generators and associated components are needed.
Some key advantages of this technology include:
Practicability. Using supercritical water as a coolant is a mature field in multiple industries, especially in the power sector.
SCWRs rely on decades of experience from water-cooled reactors.
Improved economics is possible through simpler designs, such as a direct cycle.
SCWRs have higher thermodynamic efficiency than current water-cooled nuclear reactors.
The SCWR is the only water-cooled reactor selected by the Generation-IV International Forum (GIF). This technology truly leverages the experience from their Generation I, II, and III(+) predecessors, the vast majority of which are water-cooled reactors. In essence, SCWR represents the natural evolution of water-cooled reactors.
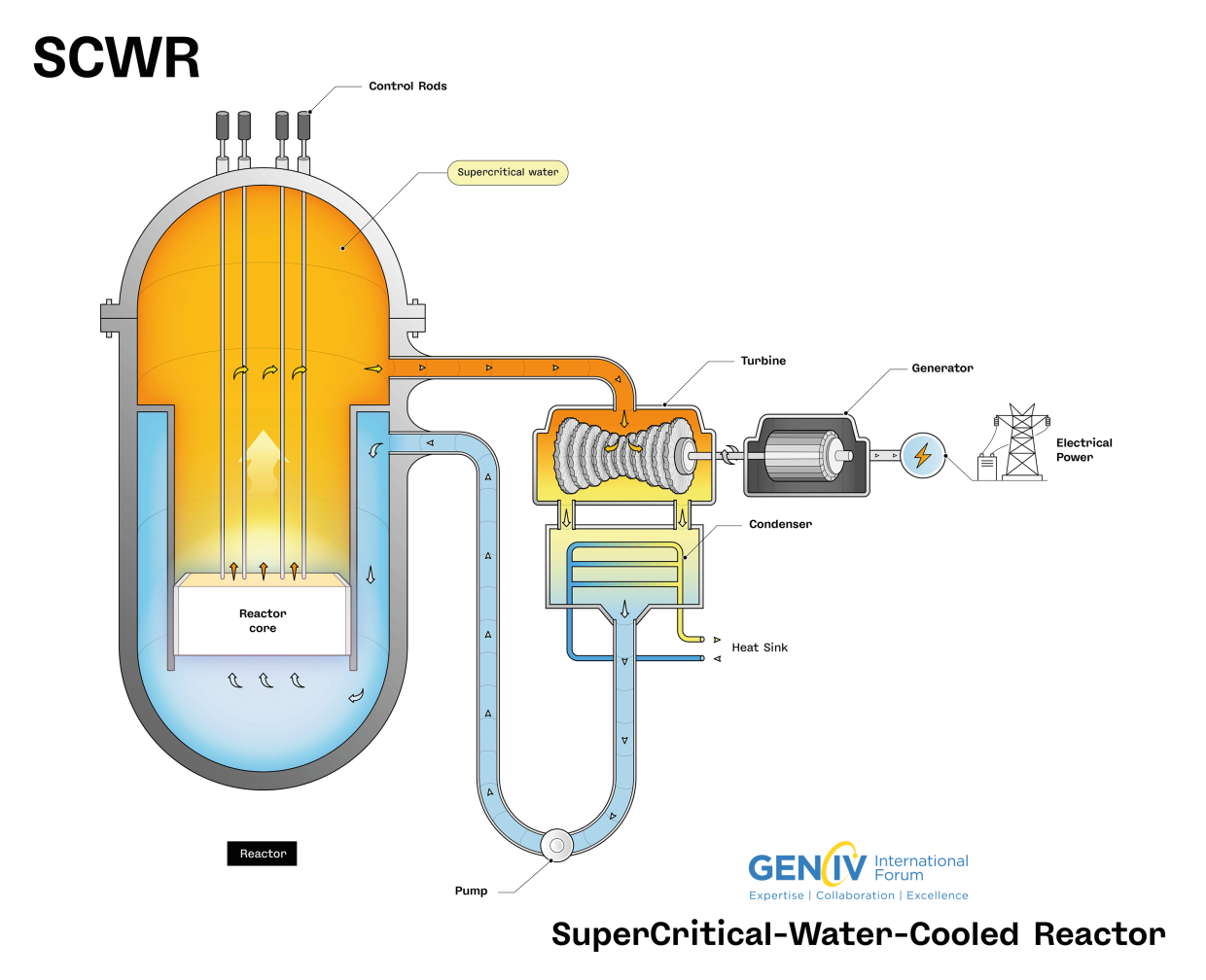
Attributes of the SCWR
The following tables present several SCWR design concepts developed worldwide. The first table is for conventional-size water-cooled reactors, and the second table presents the Supercritical water-cooled SMRs.
| Canada | China | The EU | Japan | Russian Federation | |||
---|---|---|---|---|---|---|---|---|
VVER-SKD | VVER-SCP-600 | |||||||
Type | PT | PV | PV | PV | PV | PV | PV | PV |
Spectrum | Thermal | Thermal | Mixed | Thermal | Thermal | Fast | Fast resonant | Fast resonant |
Pressure (MPa) | 25 | 25 | 25 | 25 | 25 | 25 | 24.5 | 27.5 |
Inlet Temp (°C) | 350 | 280 | 280 | 28 | 290 | 280 | 290 | 400 |
Outlet Temp (°C) | 625 | 500 | 510 | 500 | 560 | 501 | 540 | 520 |
Thermal Power | 2540 | 2300 | 3800 | 2300 | 3794 | 1602 | 3830 | 1250 |
Efficiency | 48 | 43 | 44 | 43.5 | 46 | 44 | 45 | 46.4 |
Active Core Height (m) | 5 | 3 | 4.5 | 4.2 | 4.2 | 2.4 | 4.07 | 0.8 |
Fuel | Pu-Th | UO2 | UO2/MOX | UO2 | UO2 | MOX | MOX | MOX |
Moderator | D2O | H2O | H2O/- | H2O | H2O | -/ZrH | - | ‑ |
# of Flow Passes | 1 | 2 | 2 | 3 | 1 | 2 | 2 | 1 |
Canada C-SCW-SMR | China CSR-150 | The EU ECC-SMART | GE | Japan SMR Class Super FR | Canada SuperSafe | |
---|---|---|---|---|---|---|
Type | PT | PV | PV | PT | PV | PT |
Spectrum | Thermal | Thermal | Thermal | Thermal | Fast | Thermal |
Pressure (MPa) | 25 | 25 | 25 | 30.33 - 24.13 | 25 | 25 |
Inlet Temp (°C) | 290 | 280 | 280 | 285.8 | 280 | 350 |
Outlet Temp (°C) | 450-500 | 500 | 500 | 565.5 | 500 | 625 |
Thermal Power | 800* | 375 | 290 | 728 | 650 | 670 |
Efficiency | 48 | 40 | 45 | 43.8 | 45 | |
Active Core Height (m) | 5 | 2.5 | 1.68 | 3 | 2 | unknown |
Fuel | UO2 | UO2 | UO2/MOX | UO2 | MOX | Pu-Th |
Moderator | D2O | H2O | H2O | H2O | - | D2O |
# of Flow Passes | 1 | 2 | 7 | 3 | 1 | 1 |
How do Super Critical Water Reactors meet Generation IV Criteria?
- SCWRs contribute to sustainability by utilizing a wide range of fuel options, including thorium and plutonium, which can be extracted from spent fuel from the current Light Water Reactor (LWR). This helps reduce the amount of nuclear waste and improves resource utilization.
- SCWRs have higher thermodynamic efficiency than current water-cooled reactors. Increasing this efficiency improves fuel utilization per unit of power.
- Several SCWRs concepts operate at a fast neutron spectrum, thus increasing significantly fuel utilization (these concepts are also referred to as supercritical water-cooled fast reactors).
- SCWRs offer increases in thermal efficiency relative to current-generation water-cooled reactors. The efficiency of a SCWR can approach 45% or more, compared to 34-36% for current reactors. SCWRs offer economic advantages due to their simplified design. For instance, the steam generators used in pressurized water reactors and the steam separators and dryers used in boiling water reactors can be eliminated as the supercritical coolant at the reactor outlet can be used directly in the supercritical "steam" turbines.
- The SCWR uses components that are already available in the energy sector. A big portion of the supply chain is already available. Therefore, there is no need to reach a number of sufficient reactors to develop or sustain the supply chain.
- The energy sector, primarily the oil and gas, already uses supercritical fluids to operate their equipment. The costs associated with the learning curve could be lower than shifting to a new technology.
- The higher steam enthalpy allows for decreasing the size of the turbine system and thus lowers the capital costs of the conventional island.
These general features offer the potential of lower capital costs for a given electric power of the plant and better fuel utilization, and thus a clear economic advantage compared with current light water reactors.
- The simplified design and fewer components reduce the potential points of failure, enhancing the safety and reliability of SCWRs.
- In case Zirconium (Zr) is excluded and substituted by, for example, austenitic alloys as a fuel cladding material due to high operating temperatures, this reduces hydrogen risk, improves oxidation resistance, and increases accident tolerance by minimizing exothermic reactions. This helps control temperatures better during a LOCA. However, the neutron transparency of alternative materials must be considered.
- SCWRs, particularly when using fuel compositions like a mix of thorium and plutonium, contribute to proliferation resistance by minimizing the risk of diverting nuclear materials for non-peaceful purposes.
- The closed fuel cycle and utilization of recycled plutonium contribute to physical protection by minimizing the availability of materials that could be used for nuclear weapons.
- Compared with non-water cooled reactors, such as liquid metal-cooled reactors, the SCWR has a number of general features different with respect to PR&PP.
- First, water is a clear liquid that allows optical surveillance of the fuel at any position, in the opened reactor, during fuel handling, or in the storage pool, no matter if it is fresh fuel or spent fuel. The fuel assemblies and even each fuel rod can be numbered, labelled, and thus identified without the need to remove them from the coolant or the core.
- Second, water is chemically inert in the containment, such that a leak of coolant does not cause any secondary damage, and safety systems simply need to replace the missing coolant. Compared with gas-cooled fast reactors, water stored inside the containment can provide a heat sink for at least several hours without needing outside heat removal.
- Regarding thermal SCWRs, the use of low-enriched fuel, which is less attractive for diversion, represents an additional advantage.
- Finally, as a consequence of more than 50 years of experience with more than 500 water-cooled reactors operating worldwide, methods for safeguards related to surveillance and physical protection are well-proven. Most of them can be applied directly to the SCWR.
Benefits and Applications of Super Critical Water Reactors
SCWRs are a natural evolution of current water-cooled reactors. The operating thermodynamic conditions are the main difference between current water-cooled reactors and SCWRs. SCWRs are designed to operate at a pressure above the critical point of water (22.064 MPa and 374.1°C), allowing for higher coolant temperatures without phase change. This results in increased plant efficiency. The SCWR design leads to plant simplification, which is a fundamental nature of water-cooled single-phase cooling reactors.
Most of the benefits come from the ability of SCWR concepts to meet Generation IV goals. These advantages are notably possible through their simplified designs and improved thermal efficiency compared to current Generation III (and III+) reactors. Furthermore, numerous intangible benefits are worth mentioning, such as the available supply chain and reduced learning curve for companies willing to use this technology.
Another essential attribute of the SCWR design is the ability to leverage the significant experience gained in the design, construction, and operation of conventional LWRs in the last 70+ years. Furthermore, there is plenty of experience in the energy conversion system from supercritical fossil-fired plants, which use supercritical water as a working fluid. This experience also means SCWR concepts can rely on mature, diversified supply chains.
Lastly, from a licensing perspective, SCWRs are still water-cooled reactors, and thus, this technology is closer to currently licensed reactors and relies on safety approaches that are very similar to those of other non-water-reactor technologies. This characteristic means that regulators may benefit from their extensive experience with water-cooled reactors currently operating when faced with licensing applications for SCWRs.
The range of end-use applications is broader as the SCWR operates at higher temperatures than current water-cooled reactors. Some other opportunities are supercritical fossil-fired plant replacement, Hydrogen production, industrial heat, and desalination.
Main Challenges for the Deployment of SCWR
There are several technological challenges associated with the development of the SCWR concept, particularly these are associated with materials and thermalhydraulics:
- Supercritical water creates a harsh environment for structural materials and thus significantly influences their lifetime. The most demanding conditions are for the fuel cladding. While there are numerous structural materials that can withstand the SC conditions, these usually have a large neutron capture cross-section impacting the neutron economy.
- Qualification process of advanced or newly developed structural materials, especially fuel cladding.
- Testing under simulating conditions involving neutron radiation.
- Optimization of water chemistry to limit the corrosion of structural materials and radiolysis (availability of testing devices)
- Lack of sufficient knowledge on thermalhydraulics systems operating near the critical point of water. This knowledge is needed for the start-up and shutdown of SCWRs.
- Validation of computational tools used for the conceptualization of nuclear reactors.
- Lack of neutron data for water and materials at supercritical conditions.
- Demonstration of the proposed passive safety systems.
How GIF is working to solve those challenges
GIF has established System Steering Committees (SSC) to implement the research and development (R&D) for each Generation IV Reactor Concept, with participation from GIF Members interested in contributing to collaborative R&D. Each System Steering Committee plans and integrates R&D projects contributing to the development of a system.
The SCWR SSC was established in 2006 with Canada and Euratom as first participants, later joined by Japan in 2007, Russia in 2011, and China in 2014. It now supervises two other projects, one of which is provisional. Each activity is governed by a Project Arrangement and managed by a Project Management Board (PMB). Those cover areas identified as key to moving the SCWR concept to the next stage and bringing it closer to a deployable Gen IV nuclear reactor.
To learn more about the progress made by each of these joint initiatives, please see the GIF annual reports.
- SCWR materials and chemistry (M&C), with Canada, China, and Euratom as participants, aim to:
- Conduct collaborative research on structural materials and chemistry development for the SCWR System
- Acquire the data needed to ensure the long-term behavior of candidate materials for in-core and out-of-core components, including the fuel cladding.
- Specify a water chemistry regime for testing candidate materials and, ultimately, for successfully operating a prototype SCWR.
- SCWR thermal-hydraulics and safety (TH&S), with Canada, China, and Euratom as participants, aim to:
- Conduct collaborative research on Thermal-hydraulics phenomena, such as heat transfer and hydraulic resistance near and above the critical point of water, nuclear safety, and stability of supercritical systems.
- Develop reliable and robust codes for the thermal-hydraulics analysis of the reactor core and fuel assembly.
- Perform experiments on thermo-hydraulics phenomena in laboratories and integral test loops.
- Use the experimental data to validate the computational tools (codes).
- Establish a database of experimental results.
- Benchmarking of analysis codes.
- The provisional SCWR system integration and assessment (SIA), with all SCWR SSC as participants, aims to:
- Assess R&D and systems based on conceptual designs.
- Define and refine requirements for the overall SCWR concept R&D.
- Review and integrate results from the R&D projects to assure consistency.
- Assess the system options and design tracks for conformance to Generation IV Technology Goals and other SCWR-specific requirements.
SCWR Projects History - Past projects
Valuable past experiences
In the 1960s, several SCWR concepts were proposed, such as the one proposed by General Electric (GE), which has a power of 300 MW supercritical water reactor (General Electric, 1959). This reactor was never built, but it offered insights into why this technology is relevant. The authors claim that improving the thermodynamic efficiency of a nuclear reactor allows for a better economy, provided that the costs related to safety do not offset the gains. However, most importantly, working with water offers practicability and thus can be industrialized as the current industry has vast experience of using water as a working fluid and as a coolant under both subcritical and supercritical conditions.
In 1966, a conceptual study of a supercritical reactor plant for merchant ships was proposed (Pravda M.F. and Lightner R.G., 1965). The proposed concept retains many advantages that have made pressurized water plants successful in marine applications. The concept offers significant savings in weight and size and, because of the improved thermodynamic cycle, a significant increase in plant efficiency. These features should reduce operating and capital costs. The authors also pointed out that the generation of power using supercritical steam has been adequately demonstrated in fossil-fired plants. The generation of supercritical steam in a reactor has not been demonstrated.
These early SCWR concepts converged to the same conclusion: The use of supercritical water in the oil and gas energy sector has been demonstrated. However, it has not been demonstrated in the nuclear power industry.
Multiple sources are now available to describe the relevant water-cooled reactors that support the development of SCWRs. For example, Guzonas et. al. 2018, and Schulenberg, 20xx), give an excellent literature review of early concepts that operated at superheated steam. Although these concepts did not operate above supercritical pressure, they operated above the critical temperature. The knowledge and experience gained through these reactors are fundamental from the materials' perspective. In Russia, a significant experience was acquired with the operation of nuclear-superheated steam channels in the pressure-tube BWRs at the I.V. Kuchatov Nuclear Power Station at Beloyarsk (referred to as the Beloyarsk), which operated for almost 3 decades at 500°C and 8 MPa (Guzonas et al. 2018). Two reactors (100 and 200 MWe) were installed. Unit 1 began operation in 1964, followed by Unit 2 in 1967. The dimensions and design of the two units were similar, employing boiling (evaporator) channels and superheater channels, but Unit 2 had a much-simplified flow and core arrangement compared to Unit 1. In particular, the boiling channels in Unit 1 were part of a closed loop that exchanged heat through a steam generator to produce saturated steam for the superheat channels, while Unit 2 was a direct cycle.
Early Concepts (1990s)
Interest in SCWR concepts underwent a renaissance in the 1990s. Researchers at the University of Tokyo published conceptual designs for thermal and fast-spectrum SCWRs (Oka and Koshizuka, 1993; Oka, 2000; Oka et al., 2010).
International Collaboration (2000s)
In the early 2000s, international collaboration played a significant role in advancing SCWR research. Organizations from Canada, China, Europe, Japan, and Russia actively collaborated to exchange knowledge and share research findings.
Besides the GIF SCWR PMBs, two coordinated research projects (CRP) under the IAEA were established to support the thermal-hydraulics and safety development of SCWRs. In 2007, a CRP entitled Heat Transfer Behaviour and Thermo-hydraulics Code Testing for Supercritical Water-Cooled Reactors (SCWRs) was granted. The objective of this CRP was "To establish accurate databases for thermal-hydraulics of supercritical-pressure fluids such as heat transfer, pressure drop, blowdown, natural circulation, and flow stability" (International Atomic Energy Agency, 2014).
A second IAEA CRP entitled "Understanding and Prediction of Thermohydraulic Phenomena Relevant to Supercritical Water-Cooled Reactors (SCWRs)" started in 2014 and finished in 2019. The overall objective of this CRP is (International Atomic Energy Agency, 2019).
The overall objective of the CRP is to improve the understanding of thermal-hydraulics phenomena and prediction accuracy of thermal-hydraulics parameters related to SCWRs and to benchmark numerical toolsets for SCWR thermal-hydraulics analyses.
Thomas Schulenberg and Laurence K H Leung., 2023 presented a literature review of several SCWRs conceptual designs developed during this period.
European Research (2006–2010)
A notable milestone in SCWR development was the High-Performance Light Water Reactor (HPLWR) project. The HPLWR aimed to design a pressure vessel-type SCWR with a thermal neutron spectrum. A pre-conceptual design of a pressure-vessel-type reactor with a 500°C core outlet temperature and 1000 MW electric power has been developed in Europe, as summarized by Schulenberg and Starflinger (Starflinger, J. and Schulenberg, T., 2012). The core design is based on coolant heat-up in 3 steps. Additional moderator for the thermal neutron spectrum is provided in water rods and in gaps between assembly boxes. The design of the nuclear island and the balance of the plant confirms results obtained in Japan, namely an efficiency improvement of up to 43.5% and a cost reduction potential of 20 to 30% compared with the latest boiling water reactors.
Canada's Research
Canada has been actively involved in SCWR research, with the Canadian Nuclear Laboratories (CNL, formerly Atomic Energy of Canada Limited —AECL) leading efforts in developing a pressure-tube type SCWR concept. The Canadian SCWR concept includes a direct cycle with steam reheat and aims for improved thermal efficiency. The reference core concept consists of a pressure-vessel type inlet plenum linked to a low-pressure calandria vessel, which houses 336 fuel channels. The operating pressure of the reactor is 25 MPa with a core outlet temperature of 625°C (matching the inlet conditions of the high-pressure turbines of fossil-fuel-fired thermal plants). All fuel channels are located inside a calandria vessel housing the low-pressure, low-temperature heavy-water moderator, which is cooled with active and passive cooling systems.
The fuel assembly consists of 64 elements distributed into two rings around a central flow tube. All fuel elements are inside a double-wall structure with an insulator between the two walls. Coolant from the inlet plenum is distributed into the fuel channel through openings acting as orifices to control the mass flow rate and dampen instability. The coolant flows through four nozzles into the central flow tube and downward to the bottom of the fuel assembly. The coolant reverses the flow direction and travels upward through the fuel elements to the outlet header.
The Gen IV Canadian National Program was established in 2005 to meet commitments to the GIF and support Gen IV R&D specific to Canada (Leung, L.K.H., et.al., 2018). It was separated into two phases and was managed by Natural Resources Canada (NRCan). The first phase (Phase I) focused on the basic research over three years, from April 2008 to March 2011.
Participants in this phase built their capability, developed new analytical tools, and enhanced their knowledge bases to support the development of the SCWR concept (e.g., thermal-hydraulics and safety R&D [@]). Several preliminary concepts were reviewed before deciding on a reference concept, the Canadian SCWR, for pursuing (Yetisir et al. 2016). The second phase (Phase II) focused on applied research covering four years from April 2011 to March 2015.
SCWR Current Developments & Future
Currently Operating
There are no SCWRs operating commercially or under construction. The development of SCWR technology is in the research and conceptual design phases, with various countries and organizations exploring the feasibility and potential applications of this advanced nuclear reactor concept.
Under Development
Currently, several Research and Development activities are carried out around the world. They are focused on the main challenges identified for the SCWR concept, namely:
- The chemistry of supercritical water under neutron irradiation and materials properties related to microstructural stability, embrittlement, and creep resistance.
- Corrosion of the fuel cladding candidates under dynamic conditions.
- The effect of oxide deposits on the performance and safety of the reactor.
- Flow instability of the coolant in SCWRs
- Heat transfer and hydraulic resistance near the critical point.
- Safety analyses to support design development.
Several reactor concepts are being investigated and developed as explained below:
Canada:
Canada is developing a pressure-tube-type SCWR concept with a 625°C core outlet temperature at a pressure of 25 MPa. The concept is designed to generate 1200 MW (electric). It has a modular fuel channel configuration with separate coolant and moderator. A high-efficiency fuel channel is incorporated to house the fuel assembly. The heavy-water moderator directly contacts the pressure tube, which is contained inside a low-pressure calandria vessel. In addition to providing moderation during normal operation, it is designed to remove decay heat from the high-efficiency fuel channel during long-term cooling using a passive moderator cooling system. A mixture of thorium oxide and plutonium is introduced as the reference fuel, which aligns with the GIF position paper on thorium fuel. The safety system design of the Canadian SCWR is similar to that of the ESBWR. However, introducing the passive moderator cooling system coupled with the high-efficiency channel could significantly reduce the core damage frequency during postulated severe accidents such as large-break loss-of-coolant or station black-out events.
Likewise, a supercritical water-cooled small modular reactor (Canadian SCW-SMR) is under development. It is based on the Canadian SCWR but with a smaller core length and reduced number of fuel channels. The selected operation conditions are less demanding than the ones proposed for the Canadian SCWR, with a maximum outlet coolant temperature of 450°C - 500°C. This concept leverages the experience and know-how acquired during conceptualizing the Canadian SCWR.
China:
Two conceptual SCWR designs with thermal and mixed neutron spectrum cores have been established by some research institutes in China under the framework of the Chinese national R&D projects from 2007-2012, covering some basic research projects on materials and thermohydraulics, the core/fuel design, the main system design (including the conventional part), safety systems design, reactor structure design, and fuel assembly structure design. The related feasibility studies have also been completed, showing that the design concept has promising prospects in terms of overall performance, design integration, component structure feasibility, and manufacturability.
The Nuclear Power Institute of China (NPIC) has been developing the CSR1000 (2300 MWth/100MWe), a pressure vessel type, thermal spectrum SCWR.
Besides the CSR1000, China is developing a small modular reactor cooled with supercritical water, and it is referred to as CSR150. This reactor is a scaled-down version of the CSR1000 and also builds on the experience gained through the development of the CSR1000.
Japan:
The earlier pre-conceptual core design studies for a core outlet temperature of more than 500°C in Japan (Tokyo University) assumed either a thermal neutron spectrum (Super LWR) or a fast neutron spectrum (Super FR). Both options were based on a coolant heat-up in two steps with intermediate mixing underneath the core. In Super LWR, an additional moderator for a thermal neutron spectrum is provided by feed water inside water rods. The fast-spectrum option (Super FR) uses zirconium-hydride (ZrH2) layers to enhance resonance capture of the neutrons by 238U in the blanket fuel assemblies in case of core voiding. A pre-conceptual design of safety systems for both options has been studied with transient analyses. The PWR-like RPV is combined with the BWR-like PCV. More recent designs (at Waseda University continuing based on the study at Tokyo University by the same principal researcher) adopted the core coolant heat-up in a single step. The latest research at Waseda University extends the Super FR core design studies to SMRs and the safety studies to severe accident management.
A pre-conceptual plant design, the JSCWR, with 1700 MW net electric power based on a pressure-vessel-type reactor has been studied by Toshiba Co. and has been assessed with respect to efficiency, safety and cost. The study confirms the target net efficiency of 44% and estimates a cost reduction potential of 30% compared with current pressurized water reactors. Safety features are expected to be similar to advanced boiling water reactors.
Russia:
Pre-conceptual designs of three variants of pressure vessel supercritical reactors with thermal, mixed and fast neutron spectrum have been conceptually developed in Russia, which joined the SCWR System Arrangement in 2011.
The VVER-SKD fuel assembly concept for Russian Federation's mixed spectrum SCWR is configured into a similar array to that of Japan's seed fuel assembly (i.e., 252 fuel rods in a hexagonal array). However, the design uses only one fuel assembly type with seed and fertile fuel layers alternating in each fuel rod. The seed layers contain MOX (Pu, U)O2 and the fertile layers contain depleted uranium with zirconium hydride. Eighteen absorber or zirconium hydride rods are inserted into the fuel assembly. In 2011, a new fuel assembly design was proposed. The new design provides the required water-uranium ratio and enhances the heat exchange in a rod bundle, reduces volumetric power load, and makes a warmer neutron spectrum in the core.
In addition, Russia is working on the Russian Fast-Resonant Spectrum SCWR. This concept is a variant of the VVER-SCP-600 design. The core is formed with fuel assemblies (FA) that differ by content of PuO2 in the fuel (16, 18.5, and 24 % weight), the reactor residence time, and the reactivity control. Axial (above and below core) and side blankets contain depleted uranium dioxide with a content of 0.2% 235U. The reactor core has 283 fuel assemblies, each containing 199 fuel elements and a central channel for a control rod. At the core periphery there is a side blanket with two rows of 66 and 72 fuel assemblies, respectively.
The European Union:
Based on the concept of the high-performance light water reactor (HPLWR) (Starflinger, J. and Schulenberg, T., 2012), an innovative concept of a small modular reactor is under development within the ECC-SMART (Joint European-Canadien-Chinese development of the small modular reactor technology) international project (Kykrova M., et.al., 2021). This project aims to propose a new and flexible power source with higher efficiency. Some of the main requirements on pre-concept of SCW-SMR (Small modular reactor cooled by supercritical water) were decreasing of peak cladding temperatures and ensuring the passive residual heat removal by natural convection. Due to smaller size of the core and the horizontal placement of the fuel assemblies (FA), the supercritical water is heated up in seven steps, when running through these assemblies, and the outer reflector around the core is used as mixing channels. Therefore, the coolant is mixing better which is further supported by internal water channel of FA, ensuring improved moderation. Coupled neutronics-thermal-hydraulics analyses have been performed to obtain the required power distributions with the highest power generation in the first few heat-up stages. The core models were developed with special attention to the reactivity reserve and also achievable cycle length (over 2 years without shuffling). The competitiveness of SCW-SMR is further supported by various coupled Apros - Serpent 2 calculations involving UO2 and UO2-MOX mixed cores. Based on these results the core/concept design is under an optimization process. Furthermore, several safety assessments have been conducted to ensure the basic Design Basis Accidents which are considered in the conceptualization and power distributions.
Important International Collaborations:
- ECC-SMART
The ECC-SMART Project, which began in September 2020, is oriented towards assessing the feasibility and identification of safety features of an intrinsically and passively safe small modular reactor cooled by supercritical water (SCW-SMR), taking into account specific knowledge gaps related to the future licensing process and implementation of this technology.
The main objectives of the project are to define the design requirements for the future SCW-SMR technology, to develop the pre-licensing study and guidelines for the demonstration of the safety in the further development stages of the SCW-SMR concept including the methodologies and tools to be used and to identify the key obstacles for the future SMR licensing and propose strategy for this process.
The ECC-SMART is based on international collaboration to bring together materials, thermal-hydraulic, and neutronics experts from Europe, Canada, China, and Ukraine, thereby taking maximum profit from the national research programs in the individual countries and focusing their knowledge and lessons learnt to the future licensing procedure and successful implementation of the SCW-SMR. The ECC-SMART project highly encourages students and young researchers in their careers by involving them in a huge spectrum of activities and accompanying events such as workshops, conferences, and exchange stays.
- IAEA CRP "Advancing Thermal-Hydraulic Models and Predictive Tools for Design and Operation of SCWR Prototypes"
The CRP specifically aims to advance the design tools and models (system and sub-channel codes) supported by computational fluid dynamics modeling applied to the design of SCWRs prototypes based on improved knowledge and understanding of thermal-hydraulics related phenomena such as but not limited to heat transfer, hydraulic resistance, instabilities, and corrosion effects. Achieving this scope will include the development of new prediction models and performance benchmarks on the specific phenomena.
The major goal of this CRP is to advance conceptual SCWR designs toward their prototyping and closing existing gaps in their thermal-hydraulic design.
Future
At a commercial scale, the nuclear industry is building Generation III+ water-cooled nuclear reactors. The SCWR is a Generation IV reactor; thus, it is expected to be built once the Generation III+ is completed. For that reason, no SCWRs operate commercially or are under construction. The development of SCWR technology is in the research and conceptual design phases, with various countries and organizations exploring the feasibility and potential applications of this advanced nuclear reactor concept.
GIF SCWR Related Publications
GIF has produced several reports and conducted analysis on SCWR Systems produced by cross cutting methodological working groups (Risk and Safety WG, Proliferation Resistance & Physical Protection Working Group). GIF's annual reports, technology roadmap and R&D Outlooks provide more information on the progress made by GIF's SCWR System Steering Committee and Project Management Boards.
References
A review of existing SuperCritical Water reactor concepts, safety analysis codes and safety characteristics, Pan Wu, Yanhao Ren, Min Feng, Jianqiang Shan, Yanping Huang, Wen Yang, Progress in Nuclear Energy, Volume 153, 2022
Cheng, L.-Y & Cojazzi, Giacomo & Renda, Guido & Cipiti, B. & Boyer, B & Edwards, G. & Hervieu, E. & TD, deWit & Hori, K. & Shiba, T. & Kim, H. & Nguyen, Frédéric & Hesketh, K. & Ende, B.. (2021). White Papers on Proliferation Resistance and Physical Protection Characteristics of the Six GEN IV Nuclear Energy Systems.
Caciuffo, Roberto & Fazio, C. & Guet, Claude. (2020). Generation-IV nuclear reactor systems. EPJ Web of Conferences. 246. 00011. 10.1051/epjconf/202024600011.
I.L. Pioro, R.B. Duffey, Heat transfer and hydraulic resistance at supercritical pressures in power engineering applications, ASME Press 2007.
Y. Oka, S. Koshizuka, Y. Ishiwatari, A. Yamaji, Super light water reactors and super fast reactors, Springer 2010.
S.B. Ryzhov, V.A.Mokhov, M.P.Nikitenko, A.K.Podshibyakin, I.G. Schekin, A.N. Churkin, Advanced designs of VVER reactor plant, The 8th International Topical Meeting on Nuclear Thermal-Hydraulics, Operation and Safety (NUTHOS-8), 10-14 October 2010, Shanghai, China, Paper N8P0184.
S. B. Ryzhov, P. L. Kirillov, et al., Concept of a Single-Circuit RP with Vessel Type Supercritical Water-Cooled Reactor, Proc. ISSCWR-5, Vancouver, Canada, 13-16 March 2011.
J. Kaneda, S. Kasahara, F. Kano, N. Saito, T. Shikama, H. Matsui, Material development for supercritical water-cooled reactor, Proc. ISSCWR-5, Vancouver, Canada, 13-16 March 2011.
K. Yamada, S. Sakurai, Y. Asanuma, R. Hamazaki, Y. Ishiwatari, K. Kitoh, Overview of the Japanese SCWR concept developed under the GIF collaboration, Proc. ISSCWR-5, Vancouver, Canada, 13-16 March 2011.
Y. Oka et al, Super Light Water Reactors and Super Fast Reactors (Supercritical-Pressure Light Water-Cooled Reactors), Springer Science+Business Media, 2010.
Y. Oka and H. Mori (eds.), Supercritical-Pressure Light Water Cooled Reactors,DOI 10.1007/978-4-431-55025-9_1, © Springer Japan 2014.
Y. Oka et al.," Research and development of super light water reactors and super fast reactors in Japan." Proc. 5th International Symposium on Supercritical Water-cooled Reactors, Vancouver, Canada, March 13-17, 2011).
M. Yetisir, W. Diamond, L.K.H. Leung, D. Martin and R. Duffey, Conceptual Mechanical Design for A Pressure-Tube Type Supercritical Water-Cooled Reactor, Proc. 5th International Symposium on Supercritical Water-cooled Reactors, Vancouver, Canada, 13-17 March 2011.
T. Schulenberg, J. Starflinger, High performance light water reactor – design and analyses, KIT Scientific Publishing 2012.
D. Guzonas, F. Brosseau, P. Tremaine, J. Meesungnoen, J.-P. Jay-Gerin, Water chemistry in a supercritical water–cooled pressure tube reactor, Nuclear Technology Vol. 179, 2012.
Special Issue: Journal of Nuclear Engineering and Radiation Science with selected "augmented and revised" papers from the 8th International Symposium on Supercritical Water-Cooled Reactors (ISSCWR-8), edited by Prof Thomas Schulenberg, July 2018.
June 2004, Pre-design studies of SCWR in fast neutron spectrum: evaluation of operating conditions and analysis of the behaviour in accidental situations - https://cathare.cea.fr/Lists/Publications/StructuredDisplayForm.aspx?ID=4957&ContentTypeId=0x010010995605D5DC4A16AA9AA61FBA54D1B200F308BA1E419F58438E6E39DA4B08D4C6 –
Schulenberg, Thomas, and Laurence K H Leung. "Chapter 8 - SuperCritical Water-Cooled Reactors (SCWRs). In Handbook of Generation IV Nuclear Reactors (Second Edition), edited by Igor L Pioro, Second Edition., 259–84. Woodhead Publishing Series in Energy. Woodhead Publishing, 2023. https://doi.org/https://doi.org/10.1016/B978-0-12-820588-4.00007-4.
Kryková, M., T. Schulenberg, M. Arnoult Růžičková, A. Sáez-Maderuelo, I. Otic, S. Czifrus, L. Cizelj, and G. L. Pavel. "European Research Program on Supercritical Water-Cooled Reactor." Journal of Nuclear Engineering and Radiation Science 7, no. 2 (2021): 1–5. https://doi.org/10.1115/1.4048901.
Schulenberg, Thomas, and Ivan Otic. "Concept of a Small Modular SCWR With Horizontal Fuel Assemblies." Journal of Nuclear Engineering and Radiation Science 8, no. 3 (2022): 8–10. https://doi.org/10.1115/1.4052191.
International Atomic Energy Agency, September 2014. Heat Transfer Behaviour and Thermohydraulics Code Testing for Super- critical Water Cooled Reactors (SCWRs). IAEA-TECDOC-1746, Vienna, Austria. 496 pp.
International Atomic Energy Agency, 2020. Understanding and Prediction of Thermohydraulic Phenomena Relevant to Supercritical Water Cooled Reactors (SCWRs). IAEA-TECDOC-1900, Vienna, Austria. 544 pp.
International Atomic Energy Agency, (May, 2021)., New CRP: Advancing Thermal-Hydraulic Models and Predictive Tools for Design and Operation of SCWR Prototypes (I31034).IAEA https://www.iaea.org/newscenter/news/new-crp-advancing-thermal-hydraulic-models-and-predictive-tools-for-design-and-operation-of-scwr-prototypes-i31034, accessed July 2024
Khumsa-Ang, Kittima, Alberto Mendoza, Armando Nava-Dominguez, Chukwudi Azih, and Hussam Zahlan. "Initial Multidisciplinary Study of Oxidized Chromium-Coated Zirconium Alloy for Fuel Cladding of SCW-SMR Concept: Weight-Gain and Thermal Conductivity Measurements and Coating Cost Evaluation." Coatings 13, no. 9 (2023). https://doi.org/10.3390/coatings13091648.
News and other information related to the SCWR Technology/System
News
December 2014, Supercritical Water Reactor - Fuel Qualification Test - https://cordis.europa.eu/project/id/269908/reporting/fr
Webinars and Podcasts
https://www.lastenergy.com/titansofnuclear/experts/armandonavadominguez