Lead Fast Reactors (LFR)
LFR (Lead-cooled Fast Reactor) systems are reactors cooled by liquid lead (Pb) or, in very few cases, by lead-bismuth (Pb-Bi) alloy and operating in the fast neutron spectrum at atmospheric pressure and high temperature. Many advantages of the LFR system are related to its choice of coolant: lead has a very high boiling point (up to 1743°C), favorable neutronic and radiation shielding properties as well as its benign interaction with water and air.
As other fast reactors designs, LFR uses Uranium and Plutonium in the form of Mixed Oxide Fuel (MOX) or nitride and allows for closing the fuel cycle and transuranic actinides burning thus improving fuel use efficiency and helping reduce the amount and the activity of radioactive waste generated during their operation. Challenges related to materials, chemistry control and the fuel cycle of the LFR are among the key points of focus of ongoing R&D cooperation on LFR systems.
Presentation of the Lead Fast Reactor System
Lead Fast Reactor (LFR) systems, cooled by liquid lead or a lead-bismuth alloy, operate with fast neutrons at atmospheric pressure and high temperatures. The LFR's advantages stem from its coolant choice: lead's high boiling point (up to 1743°C), beneficial neutronic and radiation shielding characteristics, and non-reactivity with water and air.
Like other fast reactors, LFRs utilize MOX fuel, combining Uranium and Plutonium, which enables the closing of the fuel cycle and the burning of transuranic actinides. This enhances fuel efficiency and reduces the volume and activity of radioactive waste. Current R&D collaborations focus on addressing LFR's material, chemical control, and fuel cycle challenges.
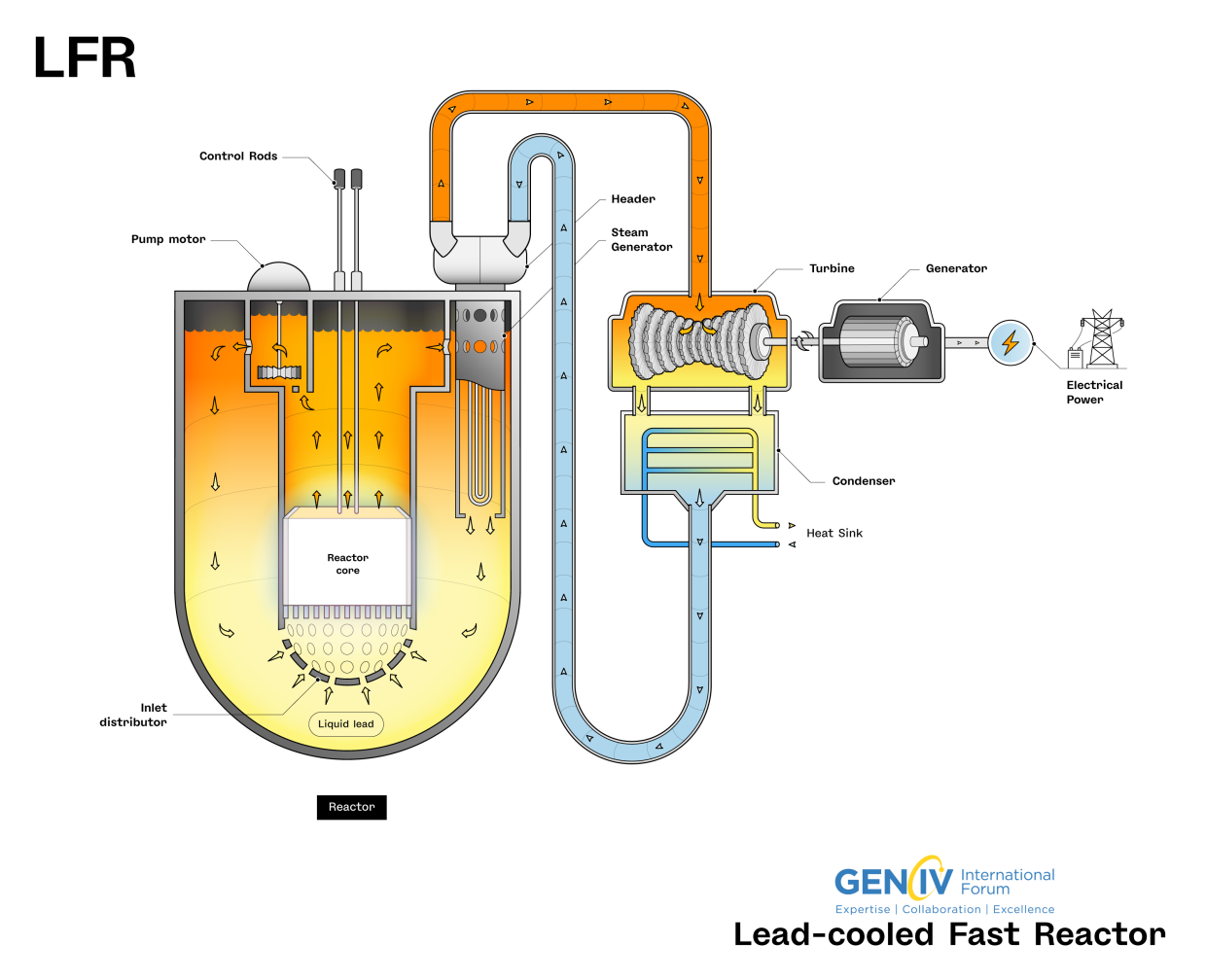
Attributes of the LFR
As of the latest update of the Generation IV Technology Roadmap, GIF considers three reference LFR concepts covering the full range of power levels, from small and intermediate to large sizes:
1) a large system rated at 600 MWe – the European lead fast reactor, intended for central station power generation;
2) a 300 MWe system of intermediate size – the Russian BREST-OD-300; and
3) a small, transportable system of 10-100 MWe size – the US small secure transportable autonomous reactor, which features a very long core life.
The expected secondary cycle efficiency of each LFR system is at or above 42%.
Reactor Parameters | Reference Value |
---|---|
Spectrum | Fast (>1 MeV) |
Core Outlet Temperature range | 480-540°C |
Coolant | Lead (Pb) / Lead-Bismuth Eutectic Alloy (Pb-Bi) |
Primary pressure | 0.1 MPa |
Power Range | 50 to 1200 MWe |
Fuel | (Pu,U) MOX (Mixed Oxide), other forms are currently being developed such as mixed nitride uranium–plutonium fuel (MNUP) for some designs |
Breeding ratio | about 1 (but design with BR>1 or BR<1 are proposed) |
How does Lead Fast Reactors meet Generation IV Criteria?
Resources utilisation:
Lead coolant neutronic properties facilitates efficient use of diverse fuel materials, achieving high conversion ratios, prolonged core life, and substantial fuel burn-up in reactors.
Waste minimization and management:
Fast neutron flux in LFR minimizes waste by enabling plutonium recycling, meeting key Generation IV conditions for waste reduction. Safe burning of recycled minor actinides further enhances the waste minimization potential of LFRs.
LFR offers cost advantages through lower capital and construction costs. Many of those gains are expected to be made in relation to the operation of the primary and atmospheric pressure and the design simplifications that are allowed by the choice of lead as a coolant.
Its higher outlet temperature, compared to conventional LWR, allows for a higher thermal efficiency and a broader scope of applications of the nuclear heat generated thus also strengthening the economics of the LFR system.
Most of the safety and reliability benefits of LFR are related to the use of liquid lead as a coolant
Lead is chemically inert and possess several attractive properties:
- The use of Pb allows for operation at atmospheric pressure
- There is no exothermic reaction between lead and water or air.
- The high boiling point of lead eliminates the risk of core voiding due to coolant boiling.
- The high density of the coolant
- facilitates insertion from the bottom of the core of control rods less dense that lead
- promotes fuel dispersion instead of compaction in case of core degradation thus minimizing re-criticality risk in partial core melt by preventing aggregation and secondary critical mass formation.
- The high heat of vaporisation and high thermal capacity of lead provide significant thermal inertia in case of loss-of-heat-sink.
- Generally speaking the high thermal inertia and negative reactivity feedback of lead systems offer, large grace times for corrective operator action.
- Lead shields gamma-rays and retains certain fission products, including iodine and caesium at temperatures up to 600°C, thereby reducing the source term in case of release of volatile fission products from the fuel.
- The low neutron moderation of lead allows greater spacing between fuel pins, leading to low core pressure drop, reduced risk of flow blockage and facilitates a high level of natural coolant circulation, which is of particular relevance in case of loss of power.
- The simple coolant flow path and low core pressure drop allow natural convection cooling in the primary system for shutdown heat removal.
- Use of lead provides also safety features such as maintaining a coolant level in case of reactor vessel leakage, thanks to leaked lead solidifying without significant adverse effects on surrounding equipment or structures
As mentioned in the conclusion of the 2021 GIF PRPP Working Group White Paper on LFR, all proposed reference LFR systems have inherent and design features favourable to PR&PP; these include the following:
- Simple, compact core;
- Low pressure operation;
- Integral power conversion equipment;
- No need of intermediate cooling system;
- Lead coolant that is chemically relatively inert and has a high margin to boiling;
- Fast spectrum that offers fuel cycle and materials management flexibility;
- Minor actinide bearing fuel;
- Natural circulation Decay Heat Removal (DHR)
Benefits and Applications of Lead Fast Reactors
LFR have many interesting safety features directly related to use of lead as a coolant. In addition, the High operating temperature at 480-520°C offers the potential to provide heat suitable for various non-electric applications (water desalination, hydrogen production, industrial processes).
In addition, LFR utilizes closed fuel cycle, in a closed fuel cycle, spent fuel is reprocessed to extract usable fuel and reduce the amount of waste generated and increase the efficient use of the energy potential contained in nuclear fuel. The extracted fuel can then be used to generate more electricity, and the remaining waste can be disposed of safely. This is an important feature of nuclear energy systems relying on a mix of thermal and fast reactors.
Main Challenges for the Deployment of LFR
Operational challenges:
High lead melting temperature:
The high melting temperature of lead (327°C) requires that the primary coolant system be maintained at temperatures to prevent the solidification of the lead coolant or at least to maintain a recirculation at the core level to allow its cooling. The use of a pool-type configuration and appropriate primary system design can provide a safe and effective resolution to this issue.
Lead opacity:
The opacity of lead, in combination with its high melting temperature, presents challenges related to in service inspection and repairs (ISI&R) and monitoring of reactor in-core components as well as fuel handling. As described in several GIF presentations, methods are under development to better tackle this challenge (cf. GIF ETWG Webinar Series 54: In-Service Inspection and Repair Developments for SFRs and Extension to other Gen-IV Systems).
Design and material challenges:
High density of lead:
The high density and corresponding high mass of lead as a coolant result in the need for careful consideration of structural design to manage seismic impacts to the reactor system.
Material selection, chemistry control to prevent corrosion:
On of the main challenges result from the tendency of lead at high temperatures to be corrosive when in contact with structural steels. This tendency, which is accelerated at higher temperatures, requires careful material and coating selection, continuous coolant chemistry control and component and system monitoring during plant operations.
How is GIF working to solve those
In its 2014 Technology Roadmap GIF identified the following areas of R&D as priorities for the LFR system:
“the main R&D efforts will be dedicated to material research, lead corrosion, coolant chemistry innovative fuel developments, and design of innovative systems and components. The following main points need further investigation:
- material corrosion;
- coolant chemistry;
- core instrumentation;
- fuel handling technology and operation;
- advanced modelling and simulation;
- fuel development: MOX for first core, then MA-bearing fuels;
- actinide management: fuel reprocessing and fabrication;
- ISI&R techniques for opaque medium;
- seismic impact.
With regard to material corrosion, the main strategy is presently based on oxygen control and/or coating/aluminisation (especially for fuel cladding). Oxygen control needs an assessment of oxygen distribution for very large pools. In parallel, the need for lead chemistry control has to be investigated while qualification under irradiation of the proposed solution must be carried out. Material studies will have to address also the combined effects of the aggressive corrosion environment and the high radiation dose to which core internal materials will be subjected. Special materials for high velocity application (i.e. mechanical primary pumps) will continue to be investigated.
To know more please consult the 2014 GIF Technology Roadmap report, accessible here.
GIF has established System Steering Committees (SSC) to implement the research and development (R&D) for each Generation IV Reactor Concept, with participation from GIF Members interested in contributing to collaborative R&D efforts on the said system. Each SSC plans and integrates R&D projects contributing to the development of a system. Each SSC is governed by a System Arrangement (SA).
In the case of LFR, a provisional SSCs has been created under a Memorandum of Understanding (MoU). Indeed, the MoU is an initial framework to allow cooperation between the interested parties and to identify areas of common interest that would justify a move to a full-fledged SSC under a SA allowing for separate projects to be carried out under Project Arrangements.
To know more about GIF activities in the area of LFRs, please consult the GIF Annual reports.
The LFR pSSC was established in 2010. Participating countries and year of joining are summarized in the table below:
Signatories to the MoU establishing the GIF Lead Cooled Fast Reactors provisional System Steering Committee (LFR pSSC) | |
---|---|
PARTNERS | JOINING DATE |
INEST (China) | October 2019 |
JRC (Euratom) | November 2010 |
Tokyo Tech (Japan) | November 2010 |
SNU (Korea) | November 2015 |
ROSATOM | July 2011 |
DOE (USA) | February 2018 |
LFR Projects History - Past projects
Soviet scientists pioneered research on heavy liquid metals (HLM) as coolants for nuclear reactors in the 1950s. This led to the successful operation of a land prototype reactor in 1959 and the deployment of Pb-Bi-cooled nuclear submarines, including the "Project 645, Submarine K-27" in 1963 and the "Projects 705 and 705K, NATO designation Alfa class" from 1971. These reactors were operating in the epithermal spectrum and cooled by a Lead Bismuth Eutectic (Pb-Bi or LBE). In total, 15 cores of this type were operated in submarine propulsion applications, the total operating time of which in all modes was about 80 reactor-years. The experience gained from the submarine nuclear reactors is valuable, but not completely transferrable to modern LFR concepts in particular due to their different operating spectrum and very peculiar characteristics and conditions of operation (very compact reactors, with highly enriched fuel and very high-power density). It is to be noted that significant decommissioning experience for these Pb-Bi propulsion reactors, even those that suffered accidents, was gained under the auspices of the Global Partnership Against the Spread of Weapons and Materials of mass destruction.
At Japan Atomic Energy Agency (JAEA) a LBE-cooled fast reactor design and the related fundamental corrosion experiments were carried out within the framework of the “Feasibility Study on Commercialized Fast Reactor Cycle Systems” from 1999 to 2005.
To evaluate the heat transfer performance of LBE in the intermediate loop and the two-phase flow characteristics of LBE, water and steam, the “CRIEPI Pb-Bi Test Loop on Thermal hydraulics” was constructed in 1997 at the Central Research Institute of Electric Power Industry (CRIEPI) in Japan. To clarify the corrosion characteristics of LBE, the “CRIEPI Static Corrosion Test Facility” was constructed in 2001.
Project/Program | Country | Power Rating | Timeframe | Notes |
---|---|---|---|---|
CRIEPI Pb-Bi Test Loop | CRIEPI, Japan | N/A | 1997 | Heat Transfer, Two-Phase Flow |
JAEA LBE-Cooled Fast Reactor | JAEA, Japan | 750 MWe (on paper, the reactor was not built) | 1999-2005 | Corrosion testing, Feasibility Study and conceptual design of the reactor |
CRIEPI Static Corrosion Test Facility | CRIEPI, Japan | N/A | 2001 | Corrosion Characteristics |
LFR Current Developments
Under Construction
There are currently no LFR in operation worldwide. The BREST-OD-300 LFR is under construction in the Russian Federation since 2021, it is serves as a demonstrator for the LFR reactor technology as well as for the demonstration of an onsite closure of the fuel cycle (with an associated fuel fabrication and a reprocessing unit it forms the project “Proryv” / Breakthrough). It is also a demonstrator that will serve as the design base for the BR-1200 LFR (of 1200MWe) to be developed in the future.
Project Name | Country | Power Rating | Expected Deployment Date | Notes |
---|---|---|---|---|
BREST-OD-300 | Russia | 700 MWth 300 MWe | Construction started in 2021 and should be finished before the end of the decade. | PuN-UN fuel |
Under Development
There is a wide range of LFR projects worldwide. Please note that their presence here does not mean those designs are endorsed by GIF and being all worked on within GIF, yet this list intends to demonstrate the activity that is ongoing worldwide around the LFR technology.
Project Name | Country | Power Rating | Expected Deployment Date | Notes |
---|---|---|---|---|
ALFRED | EU | 300 MWth | 2035-2040 | MOX Fuel Utilization |
ELFR | EU | 1500 MWth | 2040-2050 | MOX Fuel Utilization |
G4M | USA | 70 MWe | Conceptual design | UN Fuel Utilization |
MYRRHA | Belgium | 100 MWth | 2036 | MOX Fuel Utilization |
PEACER | Rep. of Korea | 300 MWe | Conceptual design | U-TRU-Zr alloy Fuel Utilization |
SEALER-55 | Blykalla (previously Leadcold), Sweden | 140 MWth | First a non nuclear demonstrator should be deployed then followed by a nuclear fueled demonstrator at the end of the decade beginning of the next. | Uranium Nitiride Fuel |
SVBR-100 | Akme Engineering, Russia | 100 MWe
| Temporarily put to hold, resumed in 2021, deployment date unknown | Lead-Bismuth cooled SMR Uranium Oxide Fuel Utilization |
Dual Fluid (DF) - 300 | Dual Fluid Energy, Canada | 600 MWth | From the NEA SMR Dashboard: “Liquid lead-cooled SMR using liquid metallic fuel with a high actinide density.” | |
LFR-AS-30 | newcleo, Italy/UK/France | 90MWth | 2030 | Use MOX Fuel. Devoted to support LFR fleet development, adopted as irradiation reactor in fast spectrum. It is part of a larger programme which include a MOX Factory construction and operation in France by 2030. |
LFR-AS-200 | newcleo, Italy/UK/France
| 480 MWth | 2033 | Uses MOX Fuel. First of a Kind. |
LFR pSSC recent Meetings
- 28th- 30th March 2023, Turin, Italy, hosted by newcleo.
- 4th- 6th October 2023, Seoul, Republic of Korea, hosted by Seoul National University.
- 22nd – 24th April 2024, Tsingdao, People's Republic of China, hosted by International Academy of Neutron Science.
- 30th of September – 3rd of October, Brasimone, Italy, hosted by ENEA (in parallel with GLANST2024)
GIF LFR Related Publications
GIF has produced several reports and conducted analysis on LFR Systems produced by cross cutting methodological working groups (Risk and Safety WG, Proliferation Resistance & Physical Protection Working Group). GIF's annual reports, technology roadmap and R&D Outlooks provide more information on the progress made by GIF's LFR provisional System Steering Committee.
GIF LFR Related Webinars
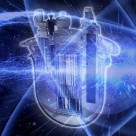
GIF talks with industry series #3 - LFR Developers: Ansaldo Nucleare
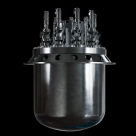
GIF talks with industry series #2 - LFR Developers: Blykalla
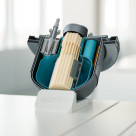
GIF talks with industry series #1 - LFR Developers: newcleo
Education and Training Series #90: Directed Energy Deposition Process of Corrosion Resistant Coating for Lead-Bismuth Eutectic Environment
Education and Training Series #23: ADVANCED LEAD FAST REACTOR EUROPEAN DEMONSTRATOR – ALFRED PROJECT
Education and Training Series #10: Lead-cooled Fast Reactor - LFR
References
The NEA SMR Dashboard Second Edition, 2024, Nuclear Energy Agency (NEA) - The NEA Small Modular Reactor Dashboard: Second Edition (oecd-nea.org)
Allen, T. R., & Crawford, D. C. (2007). Lead-Cooled Fast Reactor Systems and the Fuels and Materials Challenges. Science and Technology of Nuclear Installations, 2007, 1–11. doi:10.1155/2007/97486
Smith, C. Lead-Cooled Fast Reactor (LFR) Design: Safety, Neutronics, Thermal Hydraulics, Structural Mechanics, Fuel, Core, and Plant Design. United States: N. p., 2010. Web.
Generation IV Nuclear Energy Systems System Research Plan for the Lead-cooled Fast Reactor, GIF report
A. Alemberti et al., “Lead-cooled Fast Reactor (LFR) System Safety Assessment,” https://www.gen-4.org/gif/upload/docs/application/pdf/2020-06/gif_lfr_s…, GIF, 2020.
U.S. DOE Nuclear Energy Research Advisory Committee, Generation IV International Forum. A technology roadmap for Generation IV nuclear energy systems. USA: U.S. DOE Nuclear Energy Research Advisory Committee, Generation IV International Forum; 2002. Report No.: GIF-002-00.
GIF Policy Group. "Technology roadmap update for Generation IV nuclear energy systems", USA: The OECD Nuclear Energy Agency for the Generation IV International Forum; January 2014.
Review of Generation IV Nuclear Energy Systems, Technical Report, IRSN, 2015.
Alemberti A., Frogheri M.L., Hermsmeyer S., Smirnov L.A.V., Takahashi M., Smith C.F., et al. "Lead-cooled fast reactor (LFR) risk and safety assessment white paper, revision 8". [Access date April 2014].
Weeks J.R., "Lead, bismuth, tin and their alloys as nuclear coolants". Nucl Eng Des. 1971; 15:363–72.
Smirnov V.S., Lead-cooled fast reactor BREST—project status and prospects [presentation]. In: International Workshop on Innovative Nuclear Reactors Cooled by Heavy Liquid Metals: Status and Perspectives; 2012 Apr 17-20; Pisa, Italy.
Dragunov Y.G., Lemekhov V.V., Moiseev A.V., Tocheny L.V., Umansky A.A., “Lead-Cooled Fast-Neutron Reactor (BREST)(Approaches to the closed NFC)” Proceedings of Global 2015 September 20-24, 2015 - Paris (France) Paper 5435.
Alemberti A., Frogheri M., Mansani L., The lead fast reactor: demonstrator (ALFRED) and ELFR design [presentation]. In: International Conference on Fast Reactors and Related Fuel Cycles: Safe Technologies and Sustainable Scenarios (FR13); 2013 Mar 4-7; Paris, France.
Alemberti A., Frignani M., Villabruna G., Agostini P., Grasso G.,Turcu I., Constantin M., “ALFRED and the Lead Technology Research Infrastructure”, European research reactor Conference, RRFM2015, Bucharest, April 19-23, 2015.
Takahashi M., National status on LFR development in Japan [presentation]. In: 11th LFR Prov. SSC Meeting; 2012 Apr 16; Pisa, Italy.
Smith C.F., Halsey W.G., Brown N.W., Sienicki J.J., Moisseytsev A., Wade D.C., SSTAR: the US lead-cooled fast reactor (LFR). J Nucl Mater. 2008; 376(3):255–9.
Choi S., Hwang I.S., Cho J.H., Shim C.B., URANUS: Korean lead-bismuth cooled small modular fast reactor activities. In: Proceedings of ASME 2011 Small Modular Reactors Symposium; 2011 Sep 28–30; Washington, DC, USA. ASME Digital Collection; 2011. p. 107-112.
Wu Y.C., Bai Y.Q., Song Y., Huang Q.Y., Zhao Z.M., Hu L.Q., Development strategy and conceptual design of China lead-based research reactor. Ann Nucl Energy. 2016; 87 (Part 2):511-6.
P. Lorusso, S. Bassini, A. Del Nevo, I. Di Piazza, F. Giannetti, M. Tarantino, M. Utili, “Gen-IV LFR Development; Status & Perspectives” Progress in Nuclear Energy, 105 (2018), https://doi.org/10.1016/j.pnucene.2018.02.005
M. Caramello, M. Frignani, G. Grasso, M. Tarantino, D. Martelli, P. Lorusso, I. Di Piazza, “Improvement of ALFRED thermal hydraulics through experiments and numerical studies”, Nuclear Engineering and Design, Volume 409, 2023, 112365, ISSN 0029-5493, https://doi.org/10.1016/j.nucengdes.2023.112365
A. Alemberti, M. Caramello, M. Frignani, G. Grasso, F. Merli, G. Morresi, M. Tarantino, “ALFRED reactor coolant system design” Nuclear Engineering and Design 370 (2020), https://doi.org/10.1016/j.nucengdes.2020.110884
News related to the LFR System
January 2024 – “First lead-cooled fast neutron reactor's installation under way” – BREST OD 300 Reactor Steel base plate being installed - First lead-cooled fast neutron reactor's installation under way : New Nuclear - World Nuclear News (world-nuclear-news.org)
November 2023, Consortium to speed up development of lead-cooled SMRs - Consortium to speed up development of lead-cooled SMRs : New Nuclear - World Nuclear News (world-nuclear-news.org).
October 2023, Newcleo, Nuclear AMRC collaborate on LFR fabrication- Newcleo, Nuclear AMRC collaborate on LFR fabrication : New Nuclear - World Nuclear News (world-nuclear-news.org)
October 2022, Westinghouse and Ansaldo Nucleare collaborate on next-gen LFR nuclear plant - Westinghouse and Ansaldo Nucleare collaborate on next-gen LFR nuclear plant : New Nuclear - World Nuclear News (world-nuclear-news.org)
July 2023, Studies explore use of nuclear propulsion in shipping- Studies explore use of nuclear propulsion in shipping : Energy & Environment - World Nuclear News (world-nuclear-news.org)
May 2023, Lead-Cooled Reactor Made Tangible-Lead-Cooled Reactor Made Tangible (rosatomnewsletter.com)
March 2023, Enel to collaborate with Newcleo on LFR development - Enel to collaborate with Newcleo on LFR development : New Nuclear - World Nuclear News (world-nuclear-news.org)
June 2021, Rosatom starts construction of unique power unit with BREST-OD-300 fast neutron reactor- Rosatom starts construction of unique power unit with BREST-OD-300 fast neutron reactor - Saudi Gazette, June 10, 2021